Celestial mechanics is the study of the motions of gravitationally interacting objects, such as planets and stars. These days, people studying the subject bring powerful computers to bear on Newton's laws of motion (see Chapter 2) to understand the intricate movements of astronomical bodies. Computerized celestial mechanics enables astronomers to calculate the orbits of the planets with high precision, taking their small gravitational influences on one another into account. Even before the computer age, the discovery of one of the outermost planets, Neptune, came about almost entirely through studies of the distortions of Uranus's orbit that were caused by Neptune's gravity.
Celestial mechanics has become an essential tool as scientists and engineers navigate manned and unmanned spacecraft throughout the solar system. Robot probes can now be sent on stunningly accurate trajectories, expressed in the trade with such slang phrases as "sinking a corner shot on a billion-kilometer pool table." In fact, near-flawless rocket launches, aided by occasional midcourse changes in flight paths, now enable interplanetary navigators to steer remotely controlled spacecraft through an imaginary "window" of space just a few kilometers wide and a billion kilometers away.
Sophisticated knowledge of celestial mechanics also aids navigation of a single space probe toward several planets. For example, in 1974 the Mariner 10 spacecraft was guided into the part of Venus's gravitational field that would swing the ship around to precisely the right path for an additional trek toward Mercury. In other words, Venus itself propelled the probe in a new direction, a course alteration that required no fuel.
The accompanying figure illustrates how such a gravitational slingshot works. From the point of view of the planet, the spacecraft arrives along a hyperbolic (unbound) trajectory, passes close by, and then escapes along the same trajectory, in a new direction and with the same speed relative to the planet. However, if the planet itself is moving, some of the planet's momentum is transferred to the spacecraft as it passes by. If the orbit of the spacecraft is chosen correctly, the craft can gain energy, and speed up, as a result of the encounter. Of course, there is no "free lunch"the extra energy acquired by the spacecraft comes from the planet's motion, causing its orbit to change ever so slightly. However, since planets are so much more |
massive than spacecraft, the effect is tinyMariner 10 had no measurable effect on Venus's orbit.
Such a slingshot maneuver has been used several times. For example, the Voyager 2 spacecraft (launched in late 1977) closely passed by Jupiter (in 1979), Saturn (in 1981), Uranus (in 1986), and Neptune (in 1989). It is now moving through the outermost reaches of the solar system. The gravitational fields of the giant planets whipped the craft around at each visitation, enabling flight controllers to get considerable extra "mileage" out of the probe.
The Galileo mission to Jupiter which was launched in 1989 and arrived at its target in 1995, received three gravitational assists en routeone from Venus and two from Earth. Once in the Jupiter system, one of its probes used the gravity of Jupiter and its moons to propel it through a complex series of maneuvers designed to bring it close to all the major moons as well as to the planet itself. Every encounter with a moon had a slingshot effectsometimes accelerating and sometimes slowing the probe, but each time moving it onto a different orbitand every one of these effects was carefully calculated long before Galileo ever left Earth.
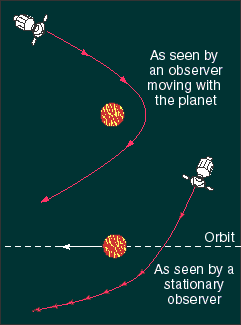
|